Remember this!
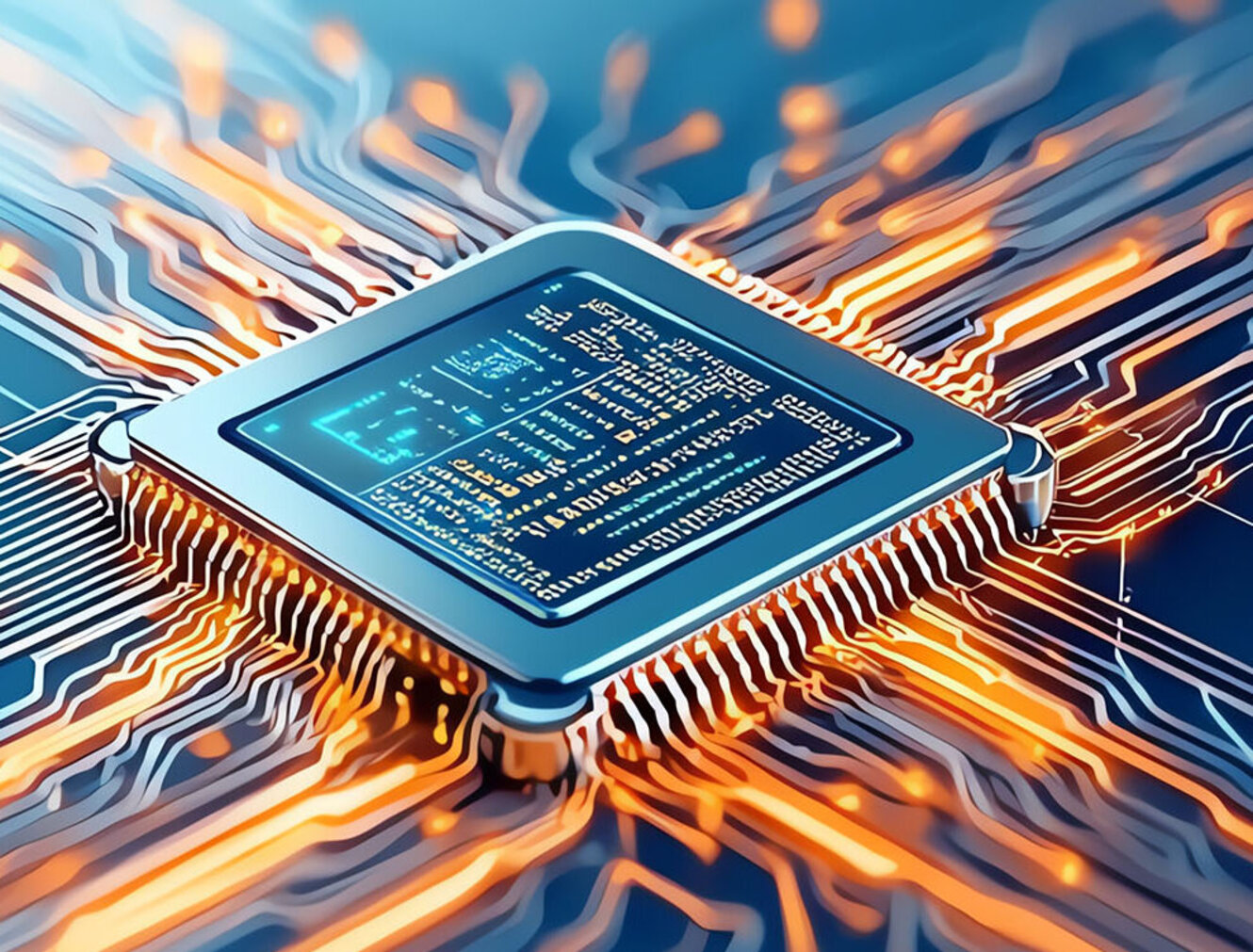
Quantum memories are essential tools for the future of quantum technology. They're needed for things like sending quantum information over long distances (quantum communication) and for building quantum computers. Traditional methods using hollow-core fibers have limitations, making it hard to create compact, efficient, and integrated quantum memories. The Nanooptics Lab (Oliver Benson) explored a new way to create these memories in cooperation with other researchers. They used tiny, 3D-printed structures called “light cages” to store light in a special kind of atomic gas (cesium). The big advantage is that many of these light cage memories can be built onto a single chip. This is important for creating more complex quantum systems. They carefully studied how well the light cages stored the light and how long they could hold onto it. If you want to know more about it, read the Quantum Memory article.
Abstract
Quantum memories are essential for photonic quantum technologies, enabling long-distance quantum communication and serving as delay units in quantum computing. Hot atomic vapors using electromagnetically induced transparency provide a simple platform with second-long photon storage capabilities. Light-guiding structures enhance performance, but current hollow-core fiber waveguides face significant limitations in filling time, physical size, fabrication versatility, and large-scale integration potential. In this work, we demonstrate the storage of attenuated coherent light pulses in a cesium (Cs) quantum memory based on a 3D-nanoprinted hollow-core waveguide, known as a light cage (LC), with several hundred nanoseconds of storage times. Leveraging the versatile fabrication process, we successfully integrated multiple LC memories onto a single chip within a Cs vapor cell, achieving consistent performance across all devices. We conducted a detailed investigation into storage efficiency, analyzing memory lifetime and bandwidth. These results represent a significant advancement toward spatially multiplexed quantum memories and have the potential to elevate memory integration to unprecedented levels. We anticipate applications in parallel single-photon synchronization for quantum repeater nodes and photonic quantum computing platforms.